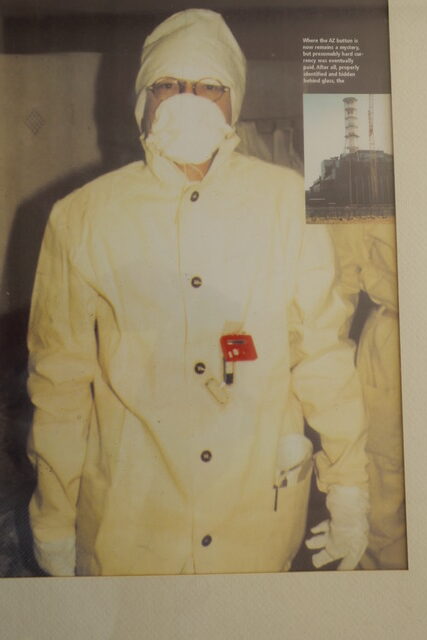
Nuclear Power: Too Big and Too Expensive
There is a story that when the Mayoress of Ipswich was invited to tour the newly built, nearby nuclear power station at Sizewell B, all the alarms went off as she was trying to leave. The beautiful green Georgian necklace she was wearing turned out to be radioactive. Better known now as ‘uranium glass’, antique jewellery made of this material can contain between 2%-25% of uranium and thus be marginally radioactive. Although many would perhaps prefer not to have such a material draped around their necks, most of it is pretty harmless. That said, UK law is absolute. Radioactive material taken into a nuclear plant becomes the property of that plant and she had to leave it behind, where it now proudly sits in the visitor’s gallery.
This may perhaps seem a little harsh, given the sheer amounts of radioactive material running around on Britain’s railways, not to mention the rest of the world, but it neatly illustrates the main problem with nuclear fission. Its primary source of energy is dangerous stuff. It is dangerous not merely at the mundane level to the people who work with it, but also on a rising scale from the Fukushima disaster to Chernobyl and beyond to Hiroshima and Nagasaki.
That said, since the Russians built the first “civil” nuclear reactor in Obninsk back in 1954, nuclear stations have been built in 30 countries numbering 439 reactors and amounting to 389.5 GW of capacity. To put this in perspective this is roughly 8.6 % of fossil fuel power capacity. Yet, instead of humming away quietly in the background, nukes seem to have an unerring ability to attract attention to themselves. Indeed, prior to climate change, anti-nuclear sentiment was a focal issue for the young: “Nuclear Power Nein Danke!” graced hundreds of handbags.
This is perhaps not a surprise. In the UK, as the Queen opened Calder Hall in 1956 and remarked about “turning swords into ploughshares”, anyone with half an eye on reality knew that the UK needed tritium and plutonium for its H-bomb programme. If this was merely a start for nuclear energy’s capacity for secrecy, when Pile No 1 caught fire just one year later, the government kept the matter under wraps. This deposited iodine-131, polonium-210, caesium-132 and a number of other radionuclides across Europe and the Irish Sea, but would have been far worse had not Sir John Cockcroft insisted on putting filters – known as Cockcroft’s follies – on top of the chimneys.
Either way, with the temperature reaching 1,300°C inside the graphite core, it quickly became apparent that putting the fire out became a matter of trial and error. They first tried CO2, then water and then finally shut off the air, under the heroic command of the deputy manager Tom Thuoy, who risked his life many times in the process and died in Australia at the age of 90, still inadequately acknowledged for his bravery. Nobody was evacuated from the area and a report on the health effects by Sir William Penney in 1958 was not made publicly available until 1988. Pile No 1 was finally dismantled in 2018.
Britain is not therefore in any position to lecture others on the subject of nuclear secrecy. Indeed, Calder Hall, renamed Windscale and subsequently Sellafield – name changes being a convenient mode of forgetting – remains a source of some embarrassment. No discredit to those who work there, but the place encapsulates one of nuclear energy’s major problems: what to do with its radioactive wastes. Do you recycle it or bury it or worse still lose it somehow? Not least of this problem is that it adds a great deal to the long-term cost of the electricity produced and nobody really knows what that cost actually is. Sure, they may be paying for it in secretive levies on the price per kWh, but nobody seems to know what to spend it on, or at least this is true in the UK.
This is not the place to go into detail about nuclear disasters, except to say that each one, regardless of the actual cause, had a pretty negative effect on the industry. The Three Mile Island loss of coolant accident in 1979 collapsed the market for new stations and 51 planned reactors were cancelled worldwide. It was 2012 before the US authorities gave permission for a new one. Chernobyl in 1986 flattened demand even further, creating a great deal more opposition, combined with ever greater safety considerations. This in turn made nukes both take much longer to build and more expensive.
For investors, whether state or private, disaster had a double whammy. Not only did an asset worth $1 billion go up in radioactive flames, but it cost as much again to clean up the mess. Furthermore, detailed scrutiny of what had gone wrong seemed to reveal that neither the operators or designers really knew what they were doing. In the case of Three Mile Island, it was a pilot operated relief valve plus maintenance checks that had covered up some switchboard lights, as well as a general catalogue of small errors and mundane design faults.
Chernobyl reactor No 4 was another matter. Ironically, the operators were engaged in a safety experiment to test whether there was enough power left in the steam turbines as the nuke was shut down to power emergency feedwater pumps in the simultaneous event of a loss of coolant and a collapse of external electricity supply. Quite why the Chernobyl operators bothered with this remains a mystery, for they had failed to make it work three times before. Either way, it all went horribly wrong. They shut down the reactor, then built it up again by removing some control rods too fast, which triggered a shut down.
Unhappily this type of reactor – the RBMK – has a ‘positive void coefficient’ which produces a localised increase in reactivity as the control rods go down. This ruptured the fuel channels. This, in turn, turned the coolant into steam and blew the lid off the building. The fire raged for eight days and 335,000 people were eventually evacuated. Sadly, those evacuees were not greeted warmly across Ukraine, Belarus and Russia on the grounds of their ‘radioactivity’. Curiously the last of the four Chernobyl reactors was only finally closed in 2000, while molten mass of radioactivity under No 4 beneath the second of its two very expensive sarcophagi is closely monitored. It is scheduled for final removal in 2065.
An emergency power source is a fundamental part of reactor design and is normally a function of the national grid. Should this be cut off, the nuke must have its own capacity. In the case of Sizewell B, this consists of four, large 8 MW diesel gen-sets conveniently located one at each corner of the building. Few accidents have quite illustrated how much this is needed as what happened to Japan’s Fukushima Daiichi reactors, on 11th March 2011. This can with some justification be described as an Act of God.
The cause of the accident was the Tohoku earthquake, which was one of the biggest ever suffered by Japan and occurred some 72 km off the east coast. Naturally, the three active reactors – 1,2 and 3 – shut down. So far so good, but what was not expected and should have been was a 14 metres-high tsunami which swept in from the sea and effectively destroyed all the plant’s emergency diesel generators. Given that the connection with the grid had been destroyed by the earthquake, the entire plant was left without power to run coolant through the still hot reactors and all three melted down.
To add insult to injury, the resultant fires and hydrogen explosions heated up the spent fuel pool of reactor no 4, adding yet further to the alarm. Those at the plant were left desperately requesting mobile power generators and batteries from wherever they could get them. Around 154,000 people were evacuated. What came from the Pacific, naturally went back into the Pacific, which included 18,000 terabecquerel of caesium-137 pretty quickly and this was still flowing in at alarming volumes two year later.
In a fish-loving nation like Japan, this was a disaster for the local fishermen, although in practice there was more danger from drinking the local milk. Either way, restrictions were rapidly put in place, although sushi-eating in California went into an abrupt decline. In any case, hindsight went into action with its usual speed. The politicians in the Diet said it was all foreseeable, while the Ministry of Economy, Trade and Industry (METI) was accused of simultaneously promoting nuclear energy, while failing to regulate it, by, of all people, the International Atomic Energy Agency in Vienna, which was also supposed to do both.
The operator, Tokyo Electric Power Company (TEPCO) was accused of not having adequate evacuation plans, while TEPCO blamed anti-nuclear protesters for this. It also came out that local TEPCO personnel were forbidden to use the phrase “meltdown” for fear of alarming the neighbours. In fact, the major flaw was having their emergency power supply too close to the sea. The really odd thing is that the earthquake and tsunami actually caused an estimated 20,000 deaths, which had nothing to do with radiation at all. Given the disaster at Fukushima where nobody was actually killed, the wider world hardly seems to have noticed this fact.
Coolant water and emergency power are thus both fundamental to the operation of nuclear energy. Water is a particular factor in a nuclear-intensive system like that of France. With 56 reactors providing 70% of its power capacity, France’s reactors have moved inland from the sea onto its magnificent rivers. In the summer of 2022 restrictions had to be placed on the plants on both the Rhone and Garonne because the coolant water was too hot to be released into the rivers, because of low water levels. This has led to concern that river-based nuclear assets may be affected by climate change itself.
This is not however the only problem faced by Electricite de France (EdF). France embraced nuclear energy with such fervour that its capacity went from 14,388 MW in 1980 to 65,125 MW in 1999. The result is that their reactors are on average 37 years old and this is beginning to show. In October 2022, half of them were shut down for maintenance, due in part to corrosion. The lights did not go out in France because the country had a lucrative electricity trade with the rest of Europe and these countries lost out instead, as did EDF’s income. This made a change from previous times in the 1990s, when overproduction sent on occasional burst of unexpected and unwanted power throughout the continental grid.
Either way, the central point about nukes is that they are not very good at “load following” as the nation switches on its electric kettles as the football match on TV reaches half-time. The best way to run a nuke is either off or on. The French did experiment with a system known as “mode G” but it all got rather complicated. The problem here in the context of climate change is that nukes provide what is effectively base load to the grid, while renewable solar and wind are highly variable. A constantly shifting supply of power from these requires an increasingly flexible “middle-order” provider that can come on line as the sun goes down and the wind falls or rises too high.
In theory, this could be provided by a lot of mini-nukes like they have in submarines. Instead of the standard size of 1,000 MW, say, you could have 10 times 100 MW ones. The problem here is that if they were dotted around the country, opposition might be high at the necessary and widespread movement of fuel and waste. Yet if they were all together and one went wrong, it might seriously affect all the others. And the probability of something going wrong would clearly be higher, while they would collectively be much more expensive to build for the same output.
This relates to EdF’s current dilemma. The current plan is to build six more large reactors, but their completion cannot come much before 2050. The question then is whether France will need this much base load power capacity, if its neighbours and France itself builds a lot of solar and wind. At least part of the problem here is that solar in particular is essentially a local, small scale, non-EdF decision. The higher the price of EdF’s electricity, the more attractive solar power investment becomes, not merely for individual houses, but for shopping malls and factories as well.
Meanwhile EdF has other bills on its way. Its nuclear waste and recycling centre at La Hague is getting pretty full as their four existing pools fill up. Short-term storage is reaching saturation point and they need to build more by 2025 at a likely cost of well over $1 billion. Furthermore, the plan is for a deep permanent repository in clay at the village of Bure in the east. The cost here, assuming that anti-nuclear protests are ignored, is put at $31 billion.
The French are not alone in this problem. The British have it as well, although on a smaller scale, but the industry does have a problem with its backside, which even if it shut down tomorrow would still be costing money for decades into the future. This is a subject to which it is worth returning as well as the hugely complex question as to the dangers of radiation to human beings. However, for now, one further point has to be made about nuclear power plant and climate change. They take a long time to build.
The standard length of time quoted at the beginning is always ‘give or take’ six years in a period of cheerful optimism. The Finns, who are no slouches when it comes to engineering, started building the Olkinoto No 3 plant in 2005. It finally started to supply the grid in 2022. In Britain, construction of the new reactor at Hinkley Point was started in 2016 with an estimate cost of £18 billion. In late 2021, it was reportedly 49% complete and the cost had risen to £23 billion. It is due on line in 2026. We shall see, but if the nuclear industry really wanted to make a difference to climate change targets in 2030, it should perhaps have started a decade ago.